A typical star is at war with itself since birth, two internal forces; gravity and fusion fighting for control but reaching an equilibrium that keeps the star stable. Nuclear fusion (not to be confused with fission) is a nuclear process where the nuclei of two atoms come together to form the nucleus of a different element in environments of high pressure and temperature, such as the core of a star. There, the immense heat causes hydrogen gas to become plasma — a state of matter where the electrons of an atom separate from the nucleus — resulting in a mix of electrons and cations (which in this case are protons since hydrogen is just an electron and a proton).

The reactions that follow are complex ones that create larger elements from hydrogen, starting with the Proton-Proton Chain reaction which converts hydrogen to helium by joining protons together (which is possible because the intense environment ‘overrides’ the proton repulsion forces) and ending with the creation of iron. Each reaction releases energy that can be seen as light and is why stars in the night sky ‘shine’.
Gravity, on the other hand, is a seemingly simple force whose nature is still being debated today. As with any object (especially those with larger masses), there is a gravitational force pushing in on the star and pressuring the core, but the outward force created by nuclear fusion prevents the star from collapsing in on itself. As long as these two forces are balanced, the star is stable.
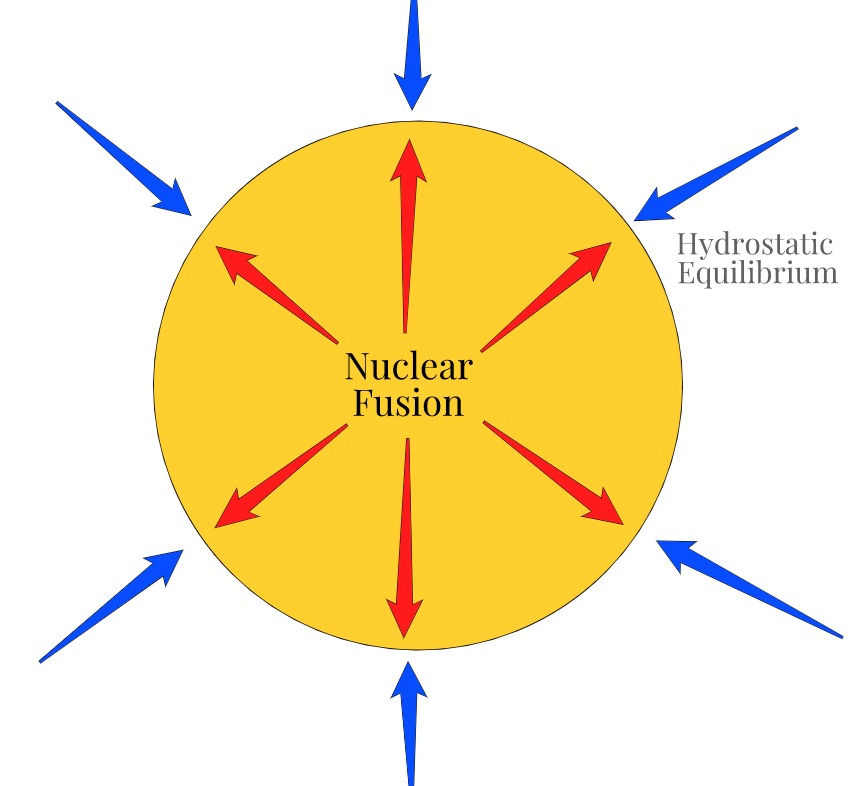
But once the core has created too much iron there isn’t enough energy to fuse it into larger elements (as iron is naturally stable and harder to break apart), so the iron just builds up in the core preventing further fusion until the star reaches a critical point where there isn’t enough nuclear fusion energy to balance the gravitational force.
It is then the star collapses and depending on the mass (the larger the mass the greater the gravitational force) it will stop at different stages.
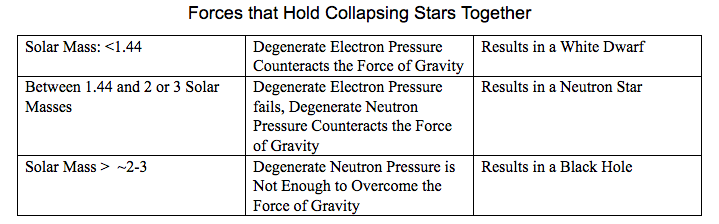
If the mass of the star is less than 1.44 solar masses (1.44 x the mass of the sun) then a quantum law known as Pauli Exclusion (PE) Principle is put in place.
No two particles with the same spin can occupy the same quantum space/energy level
(PE principle)
This results in Electron Degeneracy Pressure an outward force created by electrons pushing back against the pressure of gravity to obey the PE Principle. The degeneracy pressure is strong enough to stop the core from further collapse ending in a White Dwarf. If the mass of the star between 1.44 - 2 or 3 solar masses, the gravitational force overcomes the electron degeneracy pressure further compressing the atoms in the core, but the collapse is stopped by neutron degeneracy pressure (similar to electron degeneracy pressure and spurring from the same quantum law) and it becomes a Neutron Star. If a star is greater than 2-3 times the suns mass, both electron and neutron degeneracy pressure can’t stop the gravitational force and the core of the star collapses in on itself and becomes a black hole.
As the gravitational force overcomes the degeneracy pressures, the mass of the star is compressed into smaller areas, the radius of a White Dwarf is around 7000km, while a Neutron star is just 10km! The mass of the star stays the same, the radius just decreases causing the star to be extremely dense and have a large gravitational force.
In Einstein's space-time model of the universe, any object with mass creates a dent in the fabric of space-time and gravitational forces are a byproduct of those dents. The larger the mass of the object, the larger the dent and the greater the force of gravity. Neutron Stars have huge gravitational forces and make big warps in space-time, black holes, on the other hand, tear straight through it.
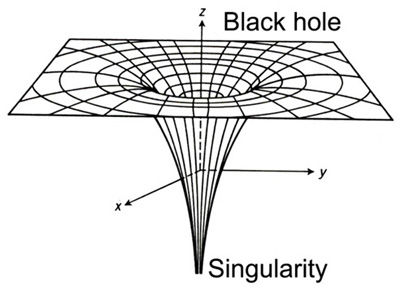
But what is a black hole? A simple definition would be an object in space-time with an escape velocity greater than the speed of light. Escape velocity is the speed one needs to be travelling to 'escape' the gravitational pull of an object. Since nothing, not even light itself can travel faster than the speed of light, this means that nothing can escape a black hole. From a space-time perspective, an object with a gravitational pull stronger than the speed of light would create a warp in the fabric of space-time so immense that it affects the way space and time themselves behave.
This distortion of time is called time-dilation, and it is not exclusive to black holes but to objects moving at high velocities (such as light) and is part of Einstein's theory of General Relativity. The light around a black hole is also severely distorted, through an effect known as gravitational lensing that we can see when we look up into the night sky. Einstein said that light always travels in accordance with space-time, so as gravity warps the fabric of space-time it should also affect the path of light. If a galaxy is in between a star and us, the warp the galaxy creates in space-time would affect the what we see. The light from the star would have to curve around the galaxy to reach us making it seem like the star is in a different position and that there are more than one stars.
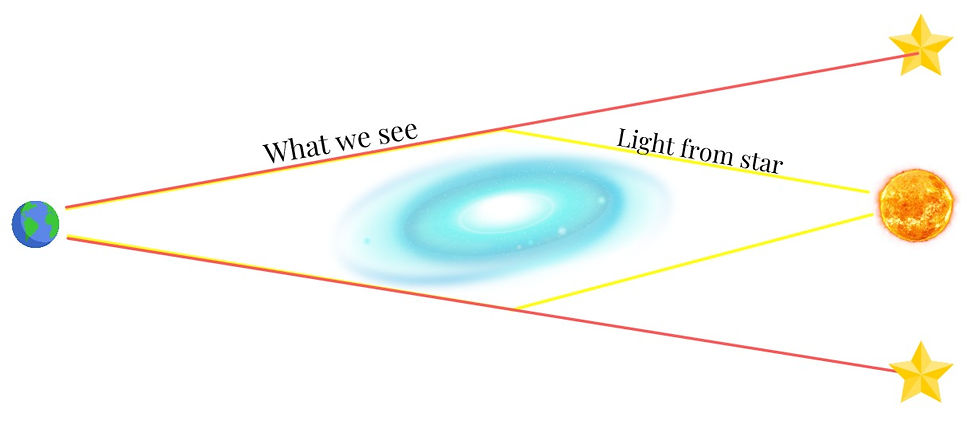
Since the gravitational warp in space-time is so immense, the light coming from a star towards a black hole would orbit the black hole a few times before continuing on its path or being sucked into the black hole itself. This area around the black hole is called the Photon Sphere.

Time, as I had mentioned, is also affected by black holes. If you shine a flashlight at the black hole, you would see the light slow down as it approaches the black hole until it finally stopped at the event horizon. Similarly, if your friend Bob was falling into a black hole while periodically flashing a light at you, as he got closer to the event horizon, the light coming from him would appear dimmer and redder. The light from the flashlight is redshifting, which means that the light needs to work harder to reach you because of the gravitational pull on it from the black hole, therefore it loses energy and its wavelength stretches out, appearing redder.

But once Bob reaches the event horizon, you would see him stop there indefinitely since the light reflecting off him can't escape past the event horizon, seeming as if time itself has stopped at the event horizon. Bob, on the other hand, would observe everything outside the event horizon as moving at the speed of light, allowing him to see the 'future' of the universe. This is due to the relativistic effects caused by the high-speed, immense gravity situations of a black hole.
A black hole has two defining factors, an event horizon and a singularity. The event horizon, in its simplest terms, is the point of no return, like in a whirlpool when you reach the place where you can no longer fight against the flow of the water. Beyond that point all matter, information and light are lost. Before the event horizon, it’s even possible to orbit a black hole as you would any other star. In fact, scientists now think there is a black hole at the centre of every galaxy. The radius of the event horizon is known as the Schwarzschild Radius, represented by;
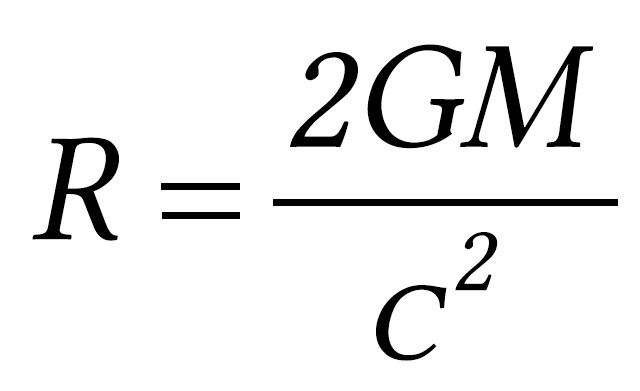
where R is the Schwarzchild radius, G is again the gravitational constant, M is the mass of the object, and c is the speed of light. It is named after Karl Schwarzschild, a physicist who derived this formula from Einstein's field equations (which describe how objects move in space-time) and it calculates the distance from the centre of a black hole to the point where nothing can escape its gravitational pull. Any object smaller than its Schwarzschild radius is a black hole, so hypothetically if you managed to squash a typical human into an area the size of an atom's nucleus or the sun to 6km across you would have a black hole.
Another defining factor is the gravitational singularity, a one-dimensional point of infinite mass, density and gravity (and therefore an infinite curvature of space-time) in an infinitely small space, the point where our laws of physics break down. When an object falls into a black hole, our current theory says that as it approaches the singularity at the speed of light, the increasing differential in gravitational attraction on different parts of the object stretches it out in a process called spaghettification. Once the object reaches the singularity, it is lost forever.
But black holes themselves are not infinite. Steven Hawking's revolutionary theory of Hawking Radiation tells us that black holes can die and that they are not at black as they may seem. Quantum theory says that particle anti-particle pairs are spontaneously formed in the vacuum of empty space before quickly annihilating each other. Hawking suggested that the gravitational pull of the Blackhole could affect the particle anti-particle pairs formed at the event horizon, and it is possible that a positive particle escapes (observed as thermal radiation or Hawking radiation) while its pair, a negative particle falls into the black hole. The negative particle eats into the mass of the black hole and after millions and millions of years, eventually, they die in a burst of energy. Yet this creates a paradox between one of the fundamental understands of the universe; that nothing, not matter, energy or even information can be created or destroyed. The information contained in every particle of matter in a black hole would be lost once the black hole dies, which is not possible according to our current laws of physics.
We are still far from understanding the mystery surrounding black holes, little is known or observed of them even today, yet on the journey to find more answers, black holes have proven to test the limits of our modern understanding of the universe, fueling progress in many areas of cosmology.
Comments